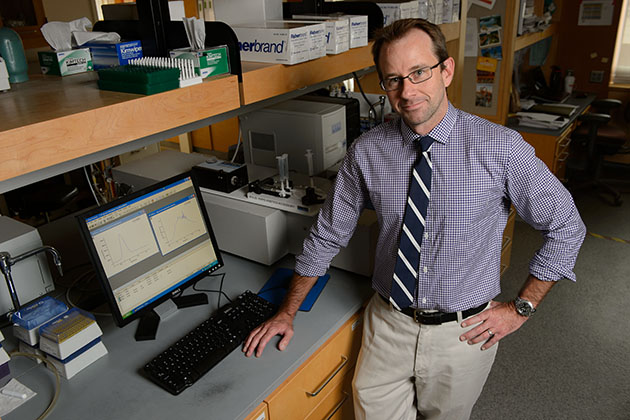
As a cell’s central power plant, the mitochondrion is a busy place.
Specially-coded proteins from the nucleus are constantly being ferried across the mitochondrion’s inner membrane, where they help the mighty organelle do its work – producing the cell’s high-energy molecules, carrying out signaling duties, and controlling cell growth.
Scientists have long known that the central channel through which most of these proteins must pass – a critical gatekeeper known as the translocase of the inner mitochondrial membrane 23 or TIM23 for short – requires an electrical field for its gating capabilities to function. But they weren’t quite sure how the whole process worked.
Until now.
Using highly sensitive fluorescent probes, a team of scientists based at UConn has managed to peer deep into the inner workings of a cell, capturing the never-before-seen structural dynamics of the TIM23 channel complex while it functioned in its natural environment.
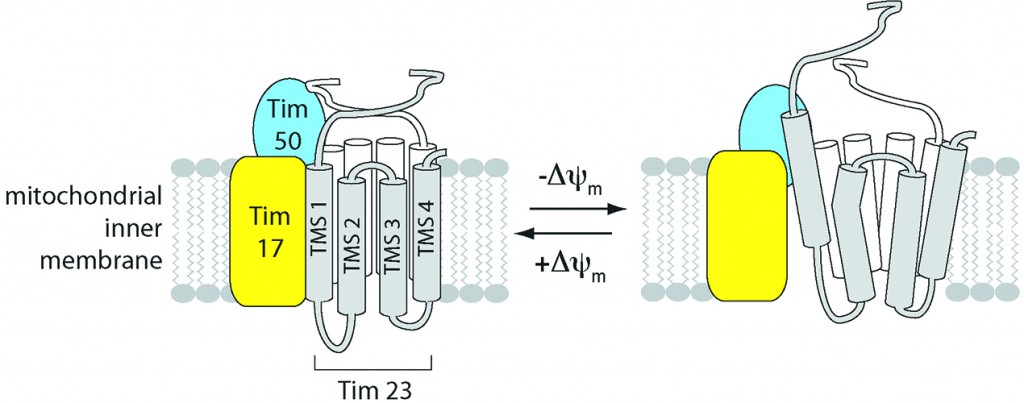
In doing so, the team, led by Nathan N. Alder, an assistant professor in the Department of Molecular and Cell Biology in the College of Liberal Arts and Sciences, discovered that the TIM23 complex not only opens and closes in response to fluctuations in the energized state of the mitochondrion’s inner membrane, as the scientific community suspected, it also changes its very structure – altering the helical shape of protein segments that line the channel – as the electrical field across the membrane drops.
The research, which appears this week in the peer-reviewed journal Nature Structural & Molecular Biology, explains how the energized state of the membrane drives the structural dynamics of membrane proteins and sheds new light on how cellular transport systems harness energy to perform their work inside the cell. It also shows how fluorescent mapping at the subcellular level may reveal new insights into the underlying causes of neurodegenerative and metabolic disorders associated with mitochondrial function.
Nikolaus Pfanner of the University of Freiburg, Germany, an international leader in the field of cellular protein trafficking, and several members of his research group, called the study “a major step towards a molecular understanding of a voltage-gated protein translocase.”
“The molecular nature of voltage sensors in membrane proteins is a central question in biochemical research,” Pfanner and his colleagues said. “The study … is not only of fundamental importance for our understanding of mitochondrial biogenesis, but also opens up new perspectives in the search for voltage-responsive elements in membrane proteins.”
Applying a new technique
The fluorescent mapping technique used in the research was a key to the project’s success. Alder says he first realized the application’s potential when he successfully mapped channel proteins in a functioning mitochondrion in 2008. In the current study, he advanced the process further, using probes to capture the behavior of a particular segment of the TIM23 channel complex as it was impacted by voltage changes in the membrane’s electrical field.
“Fluorescent mapping made this possible,” says Alder, who, as a post-doctoral student, worked with protein fluorescent labeling pioneer Arthur E. Johnson of Texas A&M’s Health Science Center. “It allowed us to peer into the functioning dynamics of a protein import channel complex that is responsible for building up the power plant of the cell … What we found was that these protein-trafficking complexes are certainly not static. This is a very, very dynamic channel.”
To monitor the fluorescence probes inside the mitochondria, the research team used advanced spectrofluorimeters equipped with xenon lamps and laser diodes to measure steady-state and time-resolved fluorescence, respectively.
To conduct the study, Alder incorporated cysteine residues modified with a fluorescent probe at specific positions along a transmembrane segment of a TIM23 complex derived from a common species of yeast, Saccharomyces cerevisiae. The team then monitored the probes in real time to observe how the channel’s voltage-gating and structure responded to induced changes in the inner membrane’s electrical field.
“It’s an indirect way of looking at the structure of something, but because we are able to look into an actually functioning mitochondrion, it’s given us a whole world of new information,” says Alder.
“That the magnitude of the voltage gradient across the membrane could play a significant role in defining the structure of these proteins is probably one of the most significant elements of this research,” he adds.
A defining moment
Watching the process was, for Alder, a defining moment in his professional career.
“When I first saw a certain kind of structure that told me I was in the middle of a channel, that was one of the most exciting times in my professional life,” he says. “I knew I was getting insight into a fundamental natural phenomenon, something no one has ever seen before.”
When Alder saw the protein-conducting channel bending and collapsing in response to changes in the membrane’s voltage levels, he was equally thrilled.
“That was one of those rare technical moments in my professional life that showed we were really getting insight into a fundamental process going on inside a cell,” he says. “It’s always been known that you need an energized membrane to make these channels work, but no one had a clue why.”
Joining Alder on the project were UConn graduate students Ketan Malhotra and Murugappan Sathappa and research associate Judith S. Landin. Johnson, Alder’s former mentor at Texas A&M, is also listed as a co-author. The work in the Alder Lab was funded by the National Science Foundation; work done in the Johnson Lab was additionally sponsored by the National Institutes of Health and the Robert A. Welch Foundation.
Alder says the next phase of the research will look toward isolating the TIM23 protein channel complex in an artificial system to see if it continues to respond to voltage fluctuations outside of its natural habitat. The research team is also hoping to identify the particular parts of the protein complex that are acting as voltage sensors.
“Once we start to identify exactly what is the voltage sensor, we will have a better understanding of the translocase process, and ultimately we can apply this knowledge to other kinds of protein transporters whose dysfunction has been implicated in the etiology of diseases such as cardiovascular disease and cancer,” Alder says. “If their function is tied to the energized state of the membrane, we’ll be able to see whether defects in that ability to couple to the membrane might be associated with the pathogenesis of these diseases.”