Creating a map of the immensely complicated structure of the brain is a major goal of neuroscience. A key challenge is that brain cells have their own, individual combinations of molecules that must be mapped as well.
UConn physiology and neurobiology Professor Linnaea Ostroff is working to revolutionize the cell mapping process. Her novel method called ultraplex microscopy takes an alternative approach to imaging, with the goal of unprecedented richness in the next generation of brain maps.
Current imaging techniques can detect only two or three molecules at a time, which limits the mapping capability of neuroscientists. One factor is the process used to prepare brain cell specimens for imaging, which degrades tissue integrity, impairs sensitivity, and employs stains that can be incompatible with different molecules, such as protein and RNA.
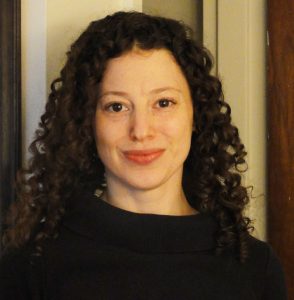
The new method developed by Ostroff involves imaging multiple molecules in the same tissue sample, a process known as multiplexing. Her ultraplex microscopy technique employs a diamond knife to subdivide specimens into ultrathin sections two orders of magnitude smaller than the cell body of a neuron.
The project, funded through a $2.24 million grant from the National Institutes of Mental Health, aims to incorporate large numbers of molecules in high-resolution microscope images of brain tissue.
“We are looking for ways to map a larger number of molecules and structures in arbitrary combinations all at the same time,” Ostroff says. “Currently, if you have a tissue specimen, you must choose what you want to do with it but you can’t do more than one thing. It’s a big limitation and it limits our understanding of the relationship of the molecules to each other. We can’t look at them together.”
Each ultrathin section produced using ultraplex microscopy is treated as a separate sample for labeling and imaging. The sections are stained and imaged individually, so that a given cell can be labeled with dozens or even hundreds of antibodies and RNA probes, in parallel, under conditions optimized for each probe.
Ultrathin sectioning is standard in electron microscopy (EM), with EM samples embedded in a hard plastic resin to allow them to be sectioned as thin as 30 nanometers. Unfortunately, EM resins block access to molecules in the tissue, and the process of embedding the samples can directly damage the molecules, especially RNA.
Ultraplex microscopy uses a new plastic resin formula that allows samples to be embedded without damage and that can then be washed off the sections to expose the molecules for labeling.
“When you embed these samples, the chemistry inherently damages the sample,” says Ostroff. “The chemistry of preparing the sample is very damaging, especially to the RNA. Ultraplex microscopy changes the chemistry used in preparing the sample. We worked out ways to preserve the sample in plastic, where all the molecules and the sample are preserved without being damaged.”
Without resin interfering, sensitive immunolabeling and RNA detection are possible, according to Ostroff. Sections are shelf-stable, so large datasets can be built up across time and even across laboratories. The goal of the project is to develop robust, reproducible protocols and workflows using prepared samples and data analysis that can be applied on a range of scales.
Ostroff’s lab specializes in electron microscopy and how synaptic changes mediate learning and memory, particularly how animals learn to differentiate between safety and danger. Memories of safety can suppress fear, anxiety, and stress responses. Understanding how they are encoded at the cellular level can shed light on the pathophysiology of anxiety disorders and post-traumatic stress disorder.
Of interest to Ostroff is how physical structure changes with experience – especially at the neuron level – and how experiences in the world physically affect the brain.
Molecules have information that lets researchers see clearly what circuits are being affected by learning. Despite advances in microscopy, researchers have yet to figure out how to localize what they see. Standard microscopes using light can see molecules but not circuits. Electron microscopy -the most powerful microscopy technique in existence, able in some cases to even visualize a single atom – can image synapses and other tiny cellular structures with perfect clarity but cannot see molecules.
“This approach bridges the two and opens up new possibilities,” says Ostroff.
UConn’s exceptional support for electron microscopy is what attracted Ostroff to the university in 2017. UConn has not one, but three electron microscope facilities, that together house more than a dozen EMs.
Ostroff’s NIMH grant is one of two she received through the agency’s BRAIN Initiative, which aims to accelerate the development and application of innovative technologies to produce a new, dynamic picture of the brain. A complementary project, funded by a $1M grant from NIMH, seeks to extend the ultraplex microscopy technique for highly detailed three-dimensional electron microscopy imaging.
Ostroff is already sharing her method with colleagues, including some in her department, and says it’s thrilling to see other researchers find ways to use it in their work. She is also sharing the technique with researchers outside UConn. The manuscript is expected to be available in the next month or so, she says.
“The anatomy of the brain is unimaginably complicated, and the field is still working hard to create tools to see what we need to see,” says Ostroff. “Our hope is that because this method does not require expensive new technology, it will allow large numbers of researchers to routinely obtain high-resolution images with molecular detail as well as facilitate collaborations across labs and over time.”