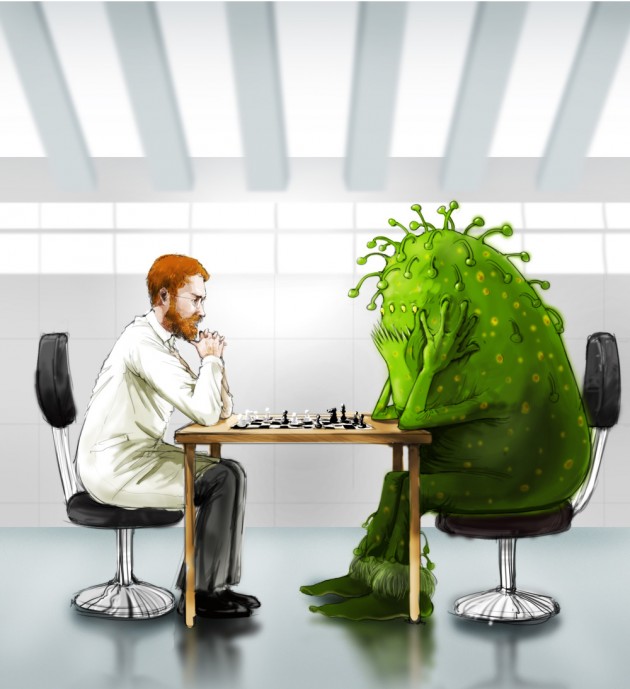
The prevalence of antibiotic-resistant bacteria has turned new drug development into a high-stakes chess game, with researchers always trying to anticipate a disease bacteria’s next move or mutation to make sure new antibiotics will be effective when they go into use.
A new software program developed by UConn pharmaceutical science professor Amy Anderson and Duke University computer scientist Bruce Donald could take some of the guesswork out of the process and help scientists bring new drugs to market faster and at a lower cost.
The program, based on a mathematical algorithm, helps researchers identify potential mutations in certain disease bacteria while new antibiotics are still in development in the lab.
“The idea is to introduce antibiotics to the clinic that are less likely to be taken out of the pipeline early because of resistance,” says Anderson, an expert in medicinal chemistry in the School of Pharmacy. “With this computer model, we can synthesize a compound that is more robust and overcomes resistance before it even gets to the clinic.”
The tool, which searches multiple amino acids – the building blocks of proteins – to find the most viable bacteria mutations, is already showing promising results.
In a study published in the Proceedings of the National Academy of Sciences, Anderson and Donald applied the algorithm to a new class of experimental antibiotics Anderson designed with UConn pharmaceutical science professor Dennis Wright to fight the potentially deadly staph infection known as methicillin-resistant Staphylococcus aureus or MRSA. The drug blocks an enzyme called dihydrofolate reductase, or DHFR, in MRSA that supports the development of drug-resistant mutations.
An estimated 80,000 cases of MRSA infection are reported in the United States each year, leading to 11,000 deaths, according to the Centers for Disease Control and Prevention. The threat of antibiotic-resistant MRSA is most profound in medical facilities, where it can cause deadly bloodstream infections, pneumonia, and surgical site infections.
The computer model predicted four mutations in the MRSA bacteria not previously known. The researchers then tested their prediction by treating a MRSA sample with the new drug compound and sequencing the DNA of the bacteria that survived. Tests confirmed the presence of two of the four genetic mutations predicted by the algorithm.
For Anderson, confirmation that the computer model worked was exciting. But she also was delighted with two additional outcomes of the study.
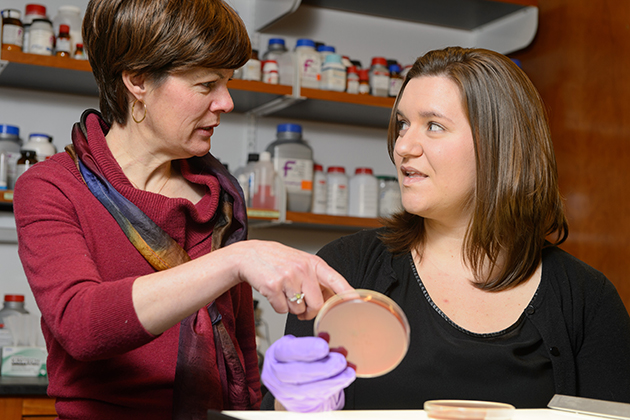
First, the study revealed that her experimental new drug was extremely resilient against antibiotic-resistant MRSA. The tests revealed that a trillion MRSA bacteria had to grow to get one initial mutation conferring resistance. But that mutation was weak and needed to be coupled with a second mutation to reach full strength. At full strength, the resistant MRSA bacteria reduced her drug’s effectiveness 58-fold. But that second mutation was also extremely rare. Together, the dual mutations appeared only once for every septillion bacteria grown. (A septillion is represented by a 1 followed by 24 zeroes).
“We learned that our compound was resistant to resistance, which is great,” says Anderson, who credits UConn doctoral student Stephanie Reeve with doing much of the grueling biochemistry behind the study. “We also now know some of the mutations that may come up, and we can now design a new generation of the compound that will be even more robust.”
The study also provided new insight into the molecular mechanics behind the genetic mutations that make some MRSA disease bacteria resistant.
“Usually, bacteria are so optimized naturally that you think of them being at the pinnacle of their evolution. So any new function that’s added may slip them off that pinnacle,” says Anderson. “But in reality, that’s not the case. The bacteria may be at a pinnacle, but they can go a little higher if they need to to survive, and these mutations help them survive. It’s fascinating.”
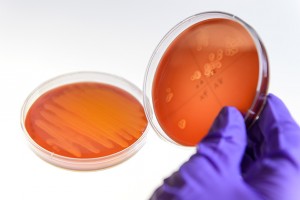
For Anderson, the most important element of the study was that the algorithm allows researchers to identify mutations proactively. Under the current system, drug developers can check a database of previously reported mutations, but the resource is limited. The existing process has hurt new drug development, which can take years, if not decades, of research and testing. Pharmaceutical companies tend to be less interested in investing in new antibiotics if they fear they are only going to last a few months in the face of bacterial resistance. If they know a new drug may be more resilient, they may be more willing to pursue it.
The computer model is not limited to MRSA bacteria, and can be applied to predict mutations in any targeted disease enzyme, Anderson says. The human immunodeficiency virus or HIV is the most well-known of these types, but the tool can also be applied to cancer agents and other viral infections.
The new drug development tool was based on earlier software developed by Donald and Anderson called OSPREY, short for Open Source Protein Redesign for You. Because it is open-source, OSPREY is freely available for other researchers to use.