Mercury is a versatile but toxic element. Though it occurs naturally at low levels, its versatility and chemical properties have led to its use in a variety of industrial applications, including the extraction of gold, despite potential danger.
It is also released into the atmosphere during fossil fuel combustion, and although many of its uses have been banned, some are still ongoing, and their industrial signatures in the form of mercury pollution linger in the environment, especially in coastal regions.
Fortunately, not all this legacy mercury pollution is “bioavailable,” or able to enter the food web, but this can change with environmental conditions, and little is known about the mechanisms that control this phenomenon. The form of mercury that is most bioaccumulative and toxic is methylmercury, which is produced from inorganic mercury in aquatic waters primarily by bacterial processes. One team of researchers has been studying the mechanisms and conditions that make methylmercury bioavailable to the organisms at the base of the marine food web and they have found that sulfur compounds, called thiols, found in natural organic matter (NOM), play a key role. Their research is published in Nature Communications.
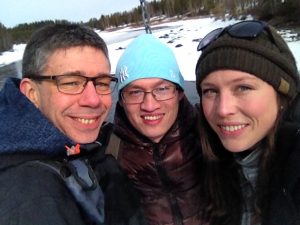
UConn Department of Marine Sciences researcher and Associate Research Professor Zofia Baumann explains that the project was started by Emily Seelen Ph.D. ’18, now a post-doctoral researcher at the University of Southern California, when she was a graduate researcher in Professor Robert Mason’s lab. Seelen worked on one of Mason’s funded projects and also received an NSF graduate fellowship that also enabled a study abroad collaboration with co-authoring chemists Erik Björn, Ulf Skyllberg, and Van Liem-Nguyen from Umeå University in Sweden, well-known for their research on mercury, who had the facilities and expertise needed for the detailed mercury and sulfur chemical analysis of samples collected in the coastal zone of the northeastern United States. Urban Wünsch from the Technical University of Denmark provided expertise in organic matter characterization.
The researchers analyzed the chemical characteristics and sulfur forms in NOM samples and performed experiments to examine the factors influencing the degree of association of methylmercury with the NOM, and the chemical factors that control the extent of the association. They also probed the mechanisms controlling the bioavailability of methylmercury in tiny organisms at the base of the food web, called phytoplankton. The experiments analyzing extracted dissolved organic matter and examining the methylmercury interactions were done in Björn’s lab, and experiments with phytoplankton uptake were performed at UConn, Baumann explains. The samples for this study were collected in conjunction with other funded research through the NIH Superfund Program which partnered Mason’s research group with Research Professor Celia Chen and other investigators at Dartmouth College, and the analysis of samples from this study provided further support for this work.
“The uptake of methylmercury from seawater into phytoplankton is the largest concentration step, because the phytoplankton act like sponges; they are so efficient,” says Baumann. “We are seeing that phytoplankton can concentrate methylmercury up to a million times, possibly more under certain conditions, compared to seawater, and every other transition up the food chain, say from phytoplankton to zooplankton, it’s never this large, more like a factor of ten.”
Baumann explains that her role was to instruct Seelen on how to perform the phytoplankton uptake experiments, drawing from her previous work tracing how contaminants, like heavy metals, move through the environment.
Water samples were collected from 20 different sites along the Eastern Coast of the United States, including estuary and open ocean sample locations. Besides measuring dissolved and particulate mercury and methylmercury in the water, characteristics such as salinity, dissolved organic carbon (DOC), and DOM were measured. The researchers suspected that methylmercury has a high affinity for sulfur, so DOM was chemically characterized in further detail to understand sulfur compounds called thiols. The chemists then performed thermodynamic and kinetic reaction experiments to understand how strongly the thiols impacted mercury’s bioavailability to phytoplankton.
Seelen explains, “A major finding was that the binding affinity of different NOM was similar across sites, and the ability of the NOM to bind methylmercury was more determined by the number of thiol groups per gram of NOM, which varied between river water and the coastal waters, where it was lowest. It was highest in salt marsh environments.”
Also, in terms of the uptake into phytoplankton, the kinetic interactions between methylmercury and NOM were rapid enough that this did not impact the methylmercury assimilation, and thus the thiol content was the major factor driving its impact on bioaccumulation.
DOM is abundant in the ocean and binds mercury very effectively, acting like a shield that prevents uptake into phytoplankton. The study showed that the offshore waters had much lower thiol content in NOM, so relatively more methylmercury is taken up by the plankton.
“We found a strong relationship between location and thiol content and were able to demonstrate that it’s very likely that the thiol contents in NOM are the key parameter controlling the availability of methylmercury to phytoplankton, rather than the NOM or DOC concentration,” says Mason.
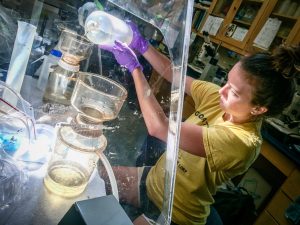
The researchers focused on estuaries and the river-estuary-coastal ocean continuum because these are areas rich in nutrients and biomass and therefore support an abundance of biological productivity, and as a result are regions where there is abundant seafood and potential for human exposure.
“This is why it’s essential to understand how mercury is cycling across these different environments,” says Björn. “We concluded that terrestrial organic matter derived from plant matter in marshes is tightly controlling methylmercury bioavailability, versus in the coastal ocean, where the NOM is derived from phytoplankton and local sources, resulting in the methylmercury being more bioavailable.”
New England is home to many estuaries historically impacted by mercury pollution and all have elevated concentrations of mercury, that are largely inorganic and not bioavailable. The same is true of Sweden and many locations around the world. Even though a small fraction of the inorganic mercury becomes bioavailable, and converted to methylmercury, the concentration is still much higher than in the open ocean. Therefore, it’s consequential to understand what’s going on in these estuaries because there is a large pool of mercury that can eventually enter the food web, and many humans rely on coastal seafood as a source of protein, and therefore are potentially exposed to elevated levels of methylmercury.
Not much can be done about the legacy mercury pollution, but future emissions can be decreased, and this is a focus of an international treaty, the Minamata Convention on Mercury. The convention came into force in 2017 after being ratified by 50 countries and there are now 147 parties to the convention, who have committed to reduce mercury use and emissions, and protect human health.
“We must remember that a major source of mercury emissions is the combustion of fossil fuel fuels here in our part of the world. In other parts of the world, small-scale, artisanal, gold mining operations contribute tremendously to the global mercury cycle,” says Baumann. “Around the world, governments must create and enforce policies, laws, and regulations to ensure that we are lowering the emissions of mercury into the atmosphere and our waters. That’s the only way that we can lower mercury in the food web in the long term. The timelines of that change are unclear and still under research.”